Research
Fate and transport of emerging contaminants in soil and groundwater
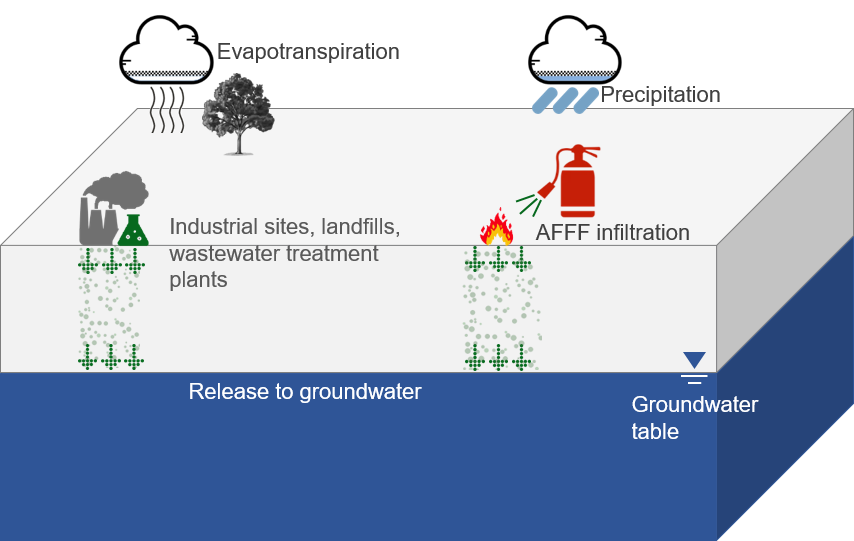
Release and migration of PFAS in the subsurface environment.
(Guo et al., 2020)
Per- and polyfluoroalkyl substances (PFAS)—a group of synthetic chemicals—have become emerging contaminants of critical concern. Since the late 1940s, PFAS have been used in a wide variety of products including nonstick coatings, textiles, paper products, and firefighting foams. Large-scale manufacturing and use of the PFAS compounds have resulted in their widespread presence in the environment. When released at the ground surface, PFAS can infiltrate into the shallow subsurface and transport through the vadose zone to contaminate groundwater. Many PFAS were created as surfactants–a property that distinguishes them from traditional contaminants—when released to the environment they tend to accumulate at air–water interfaces and may stay in the vadose zone for long periods of time. We have developed the first mathematical model that accounts for surfactant-induced flow and the complex retention processes, including air–water interfacial adsorption, for PFAS transport and migration in the vadose zone. Our computational tools can be used to investigate source zones, assess risks of contamination to groundwater, and provide guidance for developing remediation strategies.
References: Zeng & Guo (2023), Guo et al (2022), Zeng et al (2021), Zeng & Guo (2021), Guo et al (2020).Pore-scale modeling and upscaling of two-phase flow and thermodynamic phase behavior in organic-rich shale formations
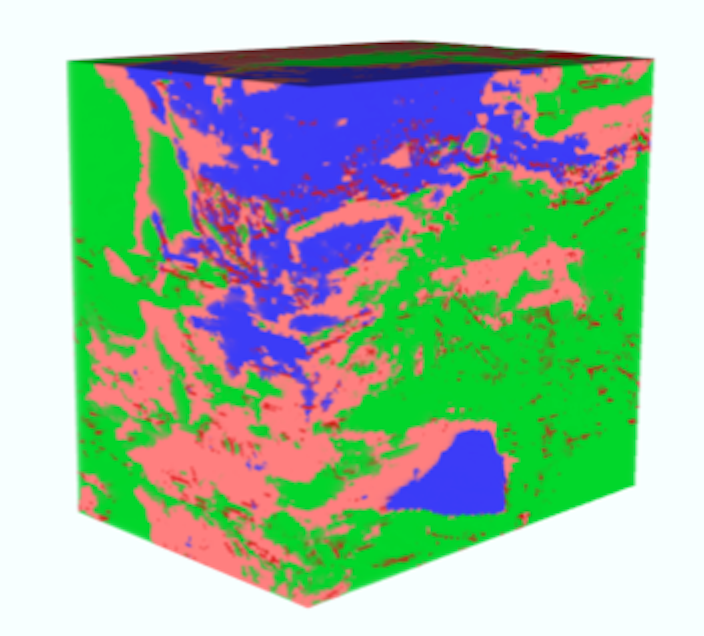
3D shale image reconstructed from FIB-SEM.
(Size: 7.22 µm x 5.11 µm x 6.82 µm)
Gas production from organic-rich shale formations has increased significantly over the past decade. However, due to the extremely small pores (in the nano-meter range) and the strong material heterogeneity, gas flow in shale is still not well understood and poses challenges for predictive reservoir-scale simulations. We are developing models for gas transport at pore scale based on 3D shale pore structures obtained by imaging techniques such as micro-/nano-CT and FIB-SEM. These models use a micro-continuum framework modified from the classic Darcy-Brinkman-Stokes equations to include both the resolved and unresolved pores. Fluid flow physics due to the very small pore sizes (e.g., slip flow, Knudsen diffusion), gas transport mechanisms in kerogen (e.g., adsorption/desorption and surface diffusion), and real gas effects can be included. We collaborate with experimentalists using shale images across a broad range of length scales and pressure decay datasets to validate our models. Our research aims to improve reservoir-scale prediction of shale gas production by providing in-depth understanding of gas flow physics at pore scale.
References: Chen et al (2021), Chen et al (2020), Guo et al (2019), Guo et al (2018).Reduced-order models for fluid injection and migration in the subsurface
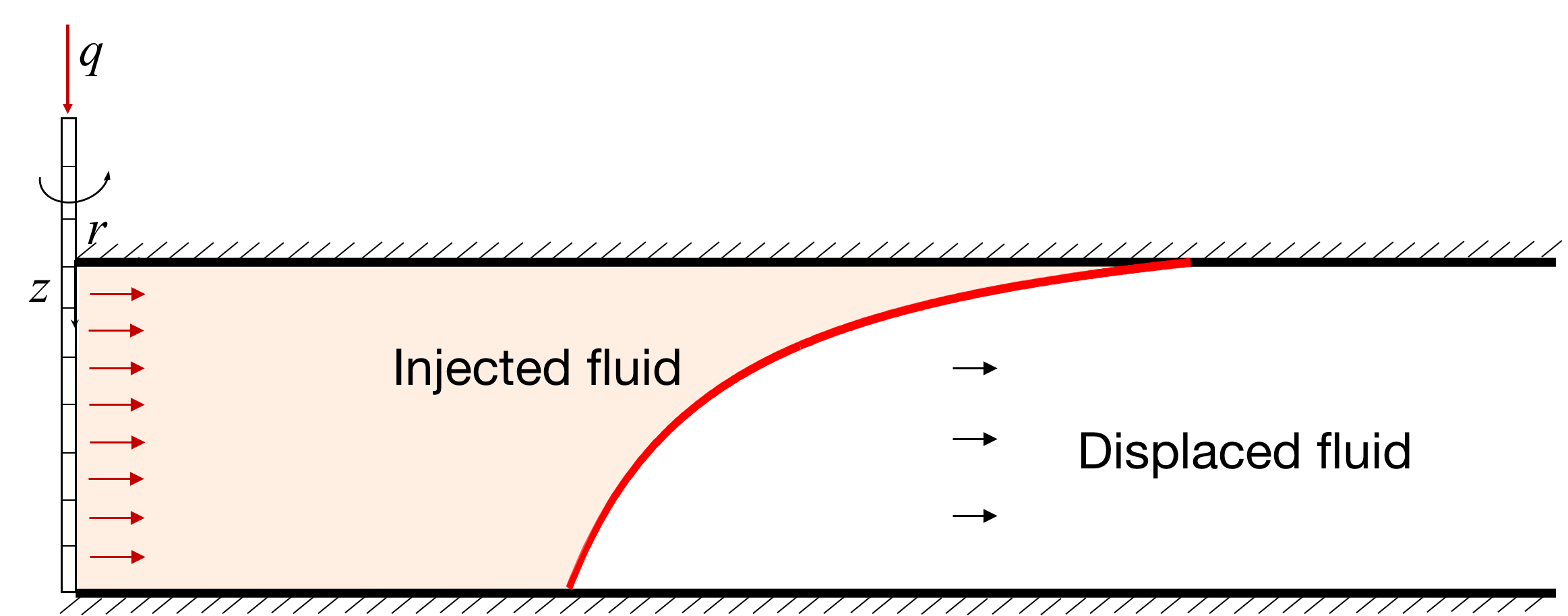
Fluid injection into a confined aquifer assuming vertical-equilibrium sharp-interface
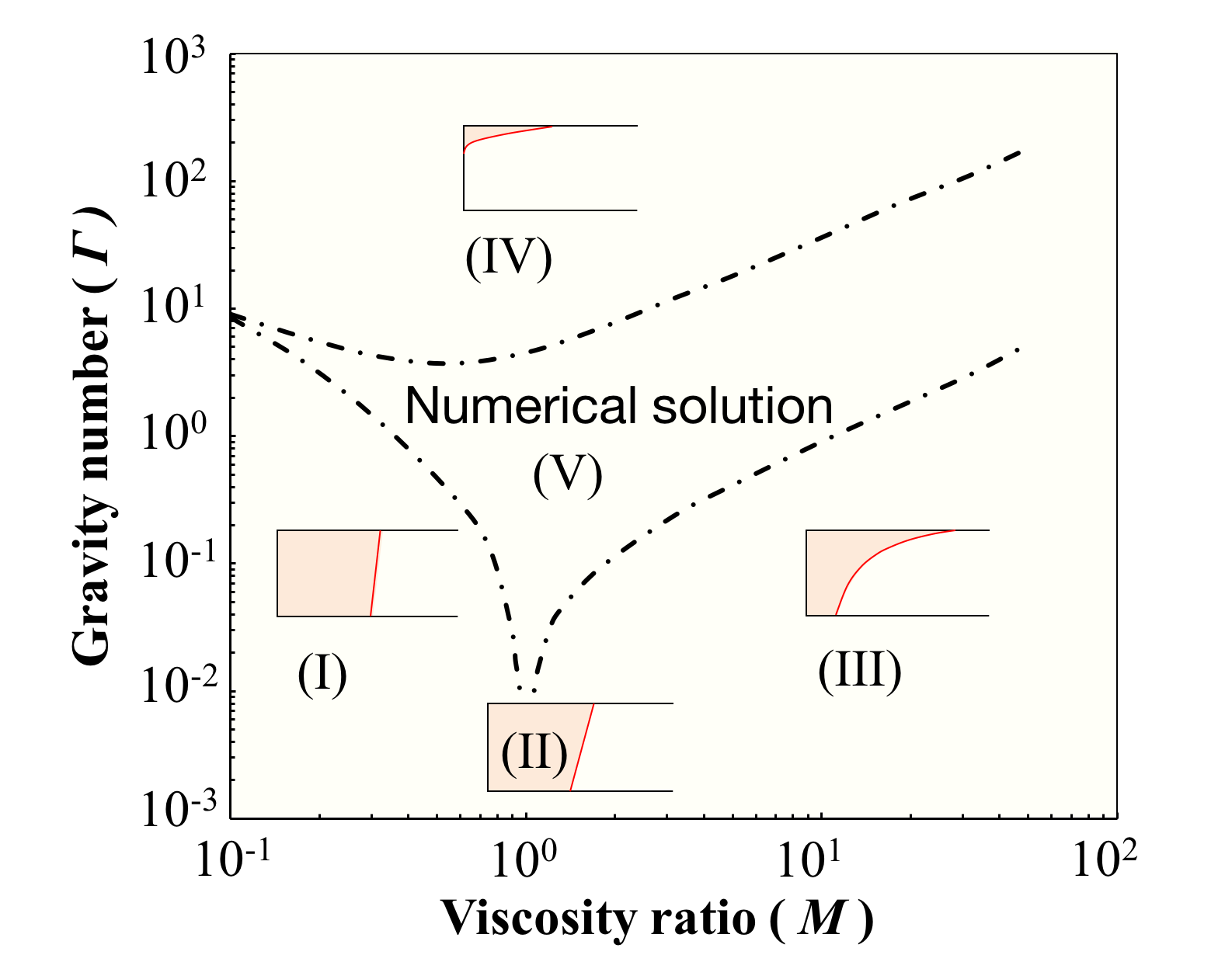
Flow regimes of fluid injection into a confined aquifer
Fluid injection into deep subsurface (e.g., enhanced oil recovery, liquid waste disposal, and geological CO2 storage) often involves strong buoyant segregation of a less-dense fluid from a more-dense fluid. When the segregation time scale is short relative to the time scale of interest, a so-called vertical equilibrium assumption may apply, which assumes the two fluids segregate rapidly and are always hydrostatic. By further assuming negligible capillary pressure between the two fluids, the two-phase flow system can be reduced to two hydrostatic fluids migrating horizontally with a macroscopic sharp fluid-fluid interface.
We use scaling and perturbation methods to analyze the reduced-order vertical-equilibrium and sharp-interface model and derive analytical solutions in four asymptotic limits. A flow regime diagram specifying the boundaries between the four flow regimes can be developed and can be used to analyze field data and parameters to identify the kinds of solutions appropriate for practical CO2 injection projects as well as other subsurface fluid injection applications. References: Tao et al (2019), Becker et al (2017), Guo et al (2016a), Guo et al (2016b).Multi-scale models for geological CO2 storage
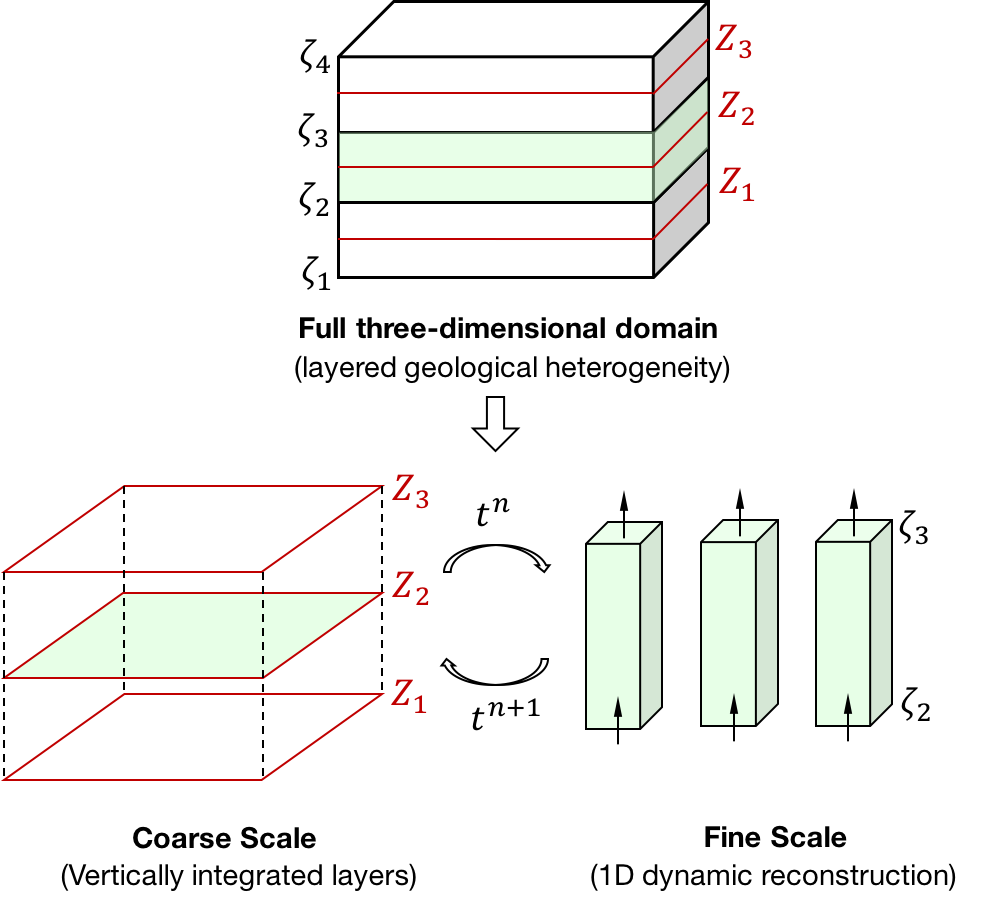
Multiscale framework for a multilayer system
Carbon Capture and Storage (CCS) has been identified as the only climate mitigation option that can significantly reduce anthropogenic CO2 emissions while allowing continued use of fossil fuels. Modeling the two-phase flow system is critical to assess storage capacity, long-term fate, and leakage risks of CO2. For some geological formations, the buoyant segregation time scale of CO2 and brine can be large where the reduced-order models fail to predict CO2 migration. To tackle this challenge while still maintaining the computational efficiency of the reduce-order models, we develop a series of multiscale models that solve the vertical dynamics of CO2 and brine as 1D fine-scale problems in a coarse-scale vertically integrated 2D framework. The capability to capture the additional two-phase flow dynamics in the vertical dimension, while maintaining much of the computational advantages of the reduced-order vertical equilibrium models makes these multiscale models very attractive for computational studies of large-scale CO2 storage systems. We continue to pursue more advanced models, with a current focus on developing a hybrid computational framework that can decompose a layered system into individual layers where each layer has the flexibility to use reduced-order model, multiscale model, or 3D model, as needed. We are also extending the reduced-order and multiscale models for CO2 storage in fractured aquifers.
References: Zheng et al (2021), Bandilla et al (2019), Guo et al (2017), Guo et al (2016), Guo et al (2014).Field-scale hybrid models for subsurface energy (methane/hydrogen) storage
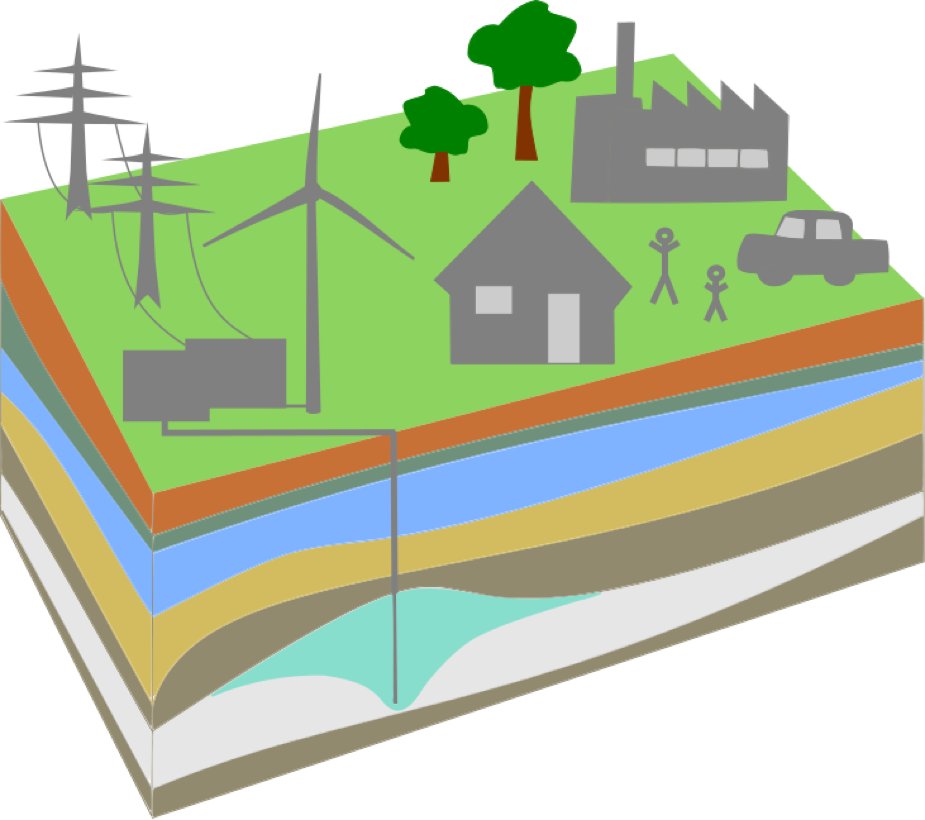
Subsurface energy storage for intermittent renewables
(Courtesy: Rainer Helmig)
.
The subsurface has recently been proposed as storage space to compensate the intermittency of renewable energy resources (e.g., solar and wind), especially in countries like Germany. This involves injecting hydrogen/methane generated from hydrolysis into the subsurface and then extract to generate energy at a later time. Daily to weekly operations due to the intermittent solar or wind resources require fast computational models to forecast gas injection/extraction and migration in the geological formations. In collaboration with Professor Rainer Helmig's group at University of Stuttgart, we are developing a hybrid modeling framework that can decompose the domain in the horizontal dimension to couple reduced-order model, multiscale model, and 3D model. The domain decomposition can be adaptive in both space and time. Together with the layered hybrid model we develop for CO2 storage, the overall hybrid modeling framework provides modeling tools for gas storage that can maintain much of the advantages of reduced-order and multiscale models, while achieving accuracy at certain spatial domain where 3D models are required.
References: Becker et al (2022). Becker et al (2018).